Humans have been building lunar landers since the 1950s, in anticipation of the first moon landing. The space industry is again planning for lunar exploration by sending astronauts and robots. However, this time the moon missions would be much different from the previous ones. These missions will need moon landers, but technologically are much more advanced than the previous ones — the capacity to carry instruments and other supplies may be for a much longer duration.
Landing on the Moon is tricky. A lander headed to the Moon can go as fast as 24,816 miles (39,937 kilometers) per hour. To land gently, these spacecraft need to slow down before touching the surface, and if there are astronauts on board, the lander needs to keep them safe, too.
Multiple factors are considered to land safely, for example: What kind of shock absorber can you make to help soften a landing. The attainment of soft landing is a difficult job in lunar exploration since the landing procedure will take place at an unprepared site and the lander may be orientated randomly with respect to the flight path.
The landers have also been designed to accommodate more mission-critical applications. This means it will be able to provide astronauts the means for conducting more advanced research. NASA has plans to colonize the Moon, and the lunar landers are supposed to set the stage for that. Thus, astronauts will need to be able to carry out geological expeditions as well as experiments to better understand the requirements to build more permanent structures on the surface of the Moon. The landers have also been designed with reusability in mind. After the first mission, the entire vehicle will be launched from the Moon to a recovery craft, allowing for multiple trips.
To prevent instability due to surface effects, the main engine cuts off at a predetermined altitude. The touchdown occurs following a short free-fall phase. The resulting kinetic energy has to be dissipated over a finite distance while providing sufficient clearance and a stable landing. At the time of impact, the lander footpad should be able to absorb shock partially and transmit the rest to the primary leg which is fitted with a shock absorber. The design of the footpad should also be such that, the lander should not topple and stay upright at the time of landing.
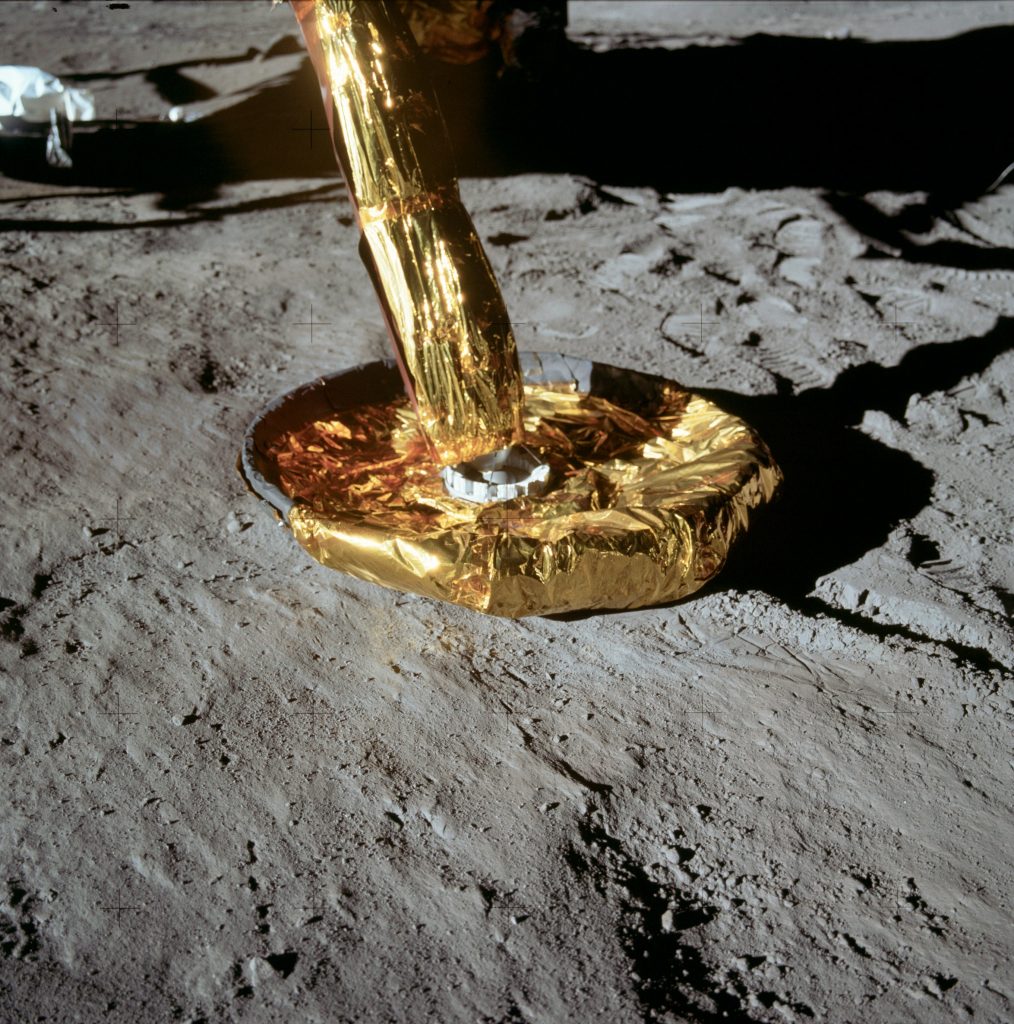
To achieve a lunar lander soft landing on the Moon’s surface, the guidance and control system plays a key role in both the descending and the landing phases.
Few more basic points are given below which must be kept in mind while designing a lander:
- Support a minimum of two crew as a sortie mission without pre-deployed assets.
- Provide a habitable environment for a few Earth days without pre-deployed assets.
- Accommodate the transfer of crew and cargo between the lander system and a crewed staging vehicle for lunar surface missions.
- Provide automated rendezvous and docking.
- Provide the capability of operating in continuous daylight conditions on the lunar surface.
- Provide the capability to perform automated transfers between lunar orbit and the lunar surface, and from the lunar surface to lunar orbit.
- Accommodate at least 100 kg of science experiments and technology demonstrations, including at least 35 kg of return mass to lunar orbit.
- Provide vehicle design and capabilities to enable effective and efficient crew performance throughout the mission.
Landing dynamics of a lander requires the understanding of the lander motion, contact dynamics, and forces interactions within the multibody system. The challenges and uncertainties in the landing dynamics and landing environment could result in a devastating outcome of the lander which could lead to a loss of mission. The landing system plays an important role in defining the stability and safety of the lunar lander. During touchdown, it provides energy absorption during landing impact and attenuates landing loads to minimize load accelerations to the lander. This could effectively prevent damages to the lander structures as well as onboard electronics. In the event of loss of control of the landing navigation system or the loss of engine control after touchdown, the landing system must be able to provide a passive method of landing the lunar vehicle without detrimental damage to the entire vehicle or toppling instability.
Some Previous Planetary Landers
Surveyor 7 Lander
Surveyor 7 lander was an unmanned vehicle with a landing mass of 305.7 kg under the American Surveyor program to achieve a successful soft landing on the Moon in 1968. The surveyor landing legs consist of aluminum tubes attached to the corners of the main body structure. Landing leg configuration consisted of one primary strut and two secondary struts attached to the landing pad. This resembled an inverted tripod landing 5 gear design type for landers. The landing leg struts and footpads contained honeycomb crushable elements for landing energy absorption. The 4.3 m footprint radius from the center of the spacecraft provides stability during landing.
Apollo 11 Lunar Module
The Apollo 11 Lunar Module (LM) was the first manned spacecraft that successfully soft-landed a crew on the Moon surface in 1969. It consisted of an ascent and descent stage, and separated from the Apollo Command/Service Module during the lunar parking orbit, and was operated by the module commander to perform the soft touchdown. The vehicle has a landing mass of 7327 kg. The landing system was of a cantilever design with the primary struts attached to the footpads and two secondary struts were connected to each of the upper sections of the primary struts. The primary struts were made of AL7178 and the secondary struts are made of AL2024. Two-staged honeycomb absorber elements are used in both the primary and secondary strut to absorb the landing impact energy. The footpad is made of AL7075 in a dish shape and the core was made of honeycomb type 2024 and 5052.
Viking 1 Lander
The Viking 1 lander was the first spacecraft that successfully achieved soft landing on Mars’s surface as part of the NASA Viking program in 1976. The Viking program consisted of an orbiter and a lander. The lander soft-landed onto Mars’s surface after separating from the orbiter during the Martian orbit. The landing system of the Viking lander was of the inverted tripod design type. It had a three-legged system which included the main strut assembly, secondary struts assembly, and a footpad for each leg. The main strut assembly contained five stages of crushable honeycomb tube core for main energy absorption. The secondary strut inboard ends were attached to load limiters which deformed upon reaching the designed limit load to protect the main body structure and onboard electronics components.
Philae Lander
Philae was the first comet lander designed to land on comet 67P/Churyumov-Gerasimenko as part of the ESA’s Rosetta mission. It was launched onboard Rosetta in 2004 and separated from Rosetta ballistically in 2014 to land on the comet surface. The lander touched down on the surface but bounced off the landing site as the harpoon system failed to anchor the lander and cold gas thrusters failed to fire upon landing. The lander came to rest in a reduced sun-lit area and had short periods of communication with Rosetta. The lander had a carbon-fiber structure and weighed approximately 98 kg. The landing system consists of three legs connected to the cardanic joint and tilt limiter assembly at the center of the body structure. With a large footprint radius, the lander was designed for great stability and minimum clearance. The landing system energy absorber was a damping mechanism that drove an electric motor and dissipated the energy through a resistor. The landing energy was also used for driving the ice screws onto the surface.
Modern Human Landing Systems (HLS)
NASA has selected three U.S. companies to design and develop human landing systems (HLS) for the agency’s Artemis program, one of which will land the first woman and next man on the surface of the Moon by 2024. NASA is on track for sustainable human exploration of the Moon for the first time in history.
The following companies were selected to design and build human landing systems:
- Blue Origin of Kent, Washington, is developing the Integrated Lander Vehicle (ILV) – a three-stage lander to be launched on its own New Glenn Rocket System and ULA Vulcan launch system.
- Dynetics (a Leidos company) of Huntsville, Alabama, is developing the Dynetics Human Landing System (DHLS) – a single structure providing the ascent and descent capabilities that will launch on the ULA Vulcan launch system.
- SpaceX of Hawthorne, California, is developing the Starship – a fully integrated lander that will use the SpaceX Super Heavy rocket.
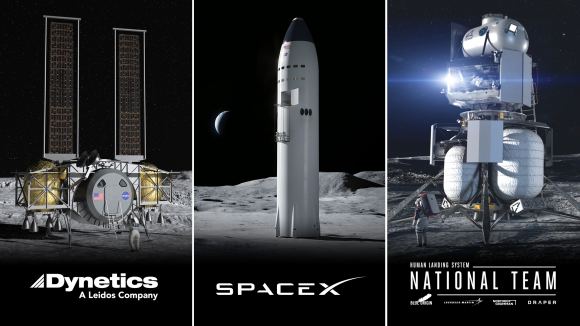
Challenges & Risks
Blue Origin | Dynetics | SpaceX |
---|---|---|
Proposed launch vehicle maturity | Proposed launch vehicle maturity | Numerous, highly complex launch, rendezvous, and refueling operations in quick succession |
Complex integrated power and propulsion systems development | Complex integrated propulsion systems development | Proposed launch vehicle maturity |
Propulsion system development complexities | Propulsion system development complexities | Complex integrated propulsion systems development |
Integrated system checkout after crew arrival | Damage to common descent-ascent vehicle from engine induced surface debris during landing | Crew egress-ingress height above the surface |
Startups for Spacecraft and Lunar Landers
Astrobotic Technology
Astrobotic Technology, Inc. is a space robotics company that seeks to make space accessible to the world. The company’s line of lunar landers and rovers delivers payloads to the Moon for companies, governments, universities, non-profits, and individuals.
The company is also developing advanced space robotics capabilities such as terrain relative navigation, mobile robotics for lunar surface operations, and reliable computing systems for mission-critical applications.
It is an American privately held company that is developing space robotics technology for lunar and planetary missions. It was founded in 2007 by Carnegie Mellon professor Red Whittaker and his associates, with the goal of winning the Google Lunar X Prize. The company is based in Pittsburgh, Pennsylvania.
The first launch of one of its spacecraft, the Peregrine lunar lander, is expected to take place in July 2021 on a Vulcan Centaur rocket.
On 11 June 2020, Astrobotic received a second contract for the CLPS program. NASA will pay Astrobotic US$199.5 million to carry the VIPER rover. Astrobotic will take NASA’s VIPER rover to the lunar surface in late 2023.
Intuitive Machines
It is a private American company headquartered in Houston, Texas. It was founded in 2013 by Steve Altemus, Kam Ghaffarian, Tim Crain to provide autonomous systems for industrial systems, drones, spacecraft, and spacesuit modeling and simulation services.
Intuitive Machines is a premier provider and supplier of space products and services that enable sustained robotic and human exploration to the Moon, Mars, and beyond. The company claims they drive markets with competitive world-class offerings synonymous with innovation, high quality, and precision. Their mission statement also includes leveraging state-of-the-art engineering tools and practices or integrating research and advanced technologies, their solutions are insightful and have a positive impact on the world.
Intuitive Machines has designed some airborne drones and spacecraft, including the Universal Reentry Vehicle (URV), the Nova-C lunar lander, and other flight instrument systems.
In November 2018, it was selected by NASA as one of the 9 companies granted the right to bid on the Commercial Lunar Payload Services program (CLPS). Their lander, Nova-C, will be proposed to NASA’s CLPS as the first lander of this program, which is focused on the exploration and use of natural resources of the Moon.
On 31 May 2019, NASA announced it had awarded Intuitive Machines $77 million to build and launch their Nova-C Moon lander.
On 13 April 2020, Intuitive Machines, under contract to carry NASA science instruments to the Moon on a privately-developed robotic spacecraft, said that its first commercial lunar mission will target landing in October 2021 near a deep, narrow valley named Vallis Schröteri.
Masten Space Systems
It is an aerospace manufacturer startup company in Mojave, California (formerly Santa Clara, California) that is developing a line of vertical takeoff, vertical landing (VTVL) rockets, initially for uncrewed research sub-orbital spaceflights and eventually intended to support robotic orbital spaceflight launches.
In 2020, NASA awarded Masten a contract for a lunar lander mission; NASA is to pay Masten 75.9 million USD for Masten to build and launch a lander called XL-1 to take NASA and other customer payloads to the south pole of the Moon. This mission will be Masten’s first space flight; it is scheduled for late 2022.
They call themselves a tight confederation of space pioneers, thought leaders, rocket scientists, dreamers, and business heavyweights, they have been wrangling rocket-powered landing from sci-fi into reality for more than 15 years.
Masten Space Systems was born in the Mojave desert in 2004 by their founder and Chief Technology Officer, David Masten, with one idea in mind:
Tear down the barriers to space, partner up with like-minded, passionate space pioneers to create value in the space ecosystem, and bring the benefits of space to humans here on Earth.
They state their mission statement as:
“Lower the barriers to space through reusability and enabling technology of entry descent and landing technologies (EDL) to ensure precise and safe landings on planets and other celestial bodies in our solar system.
Since 2004, we have been committed to rapid reusability. Our demonstrated reusability has enabled us to reliably and safely test new technologies aboard our rocket-powered landers. We believe that rockets should operate more like airplanes than ballistic missiles.
This simple mission is our contribution to a shared strategic goal of extending human presence across the solar system.”
ispace Inc.
It is a private Japanese company developing robotic spacecraft technologies to build landers and rovers to compete for both transportation and exploration mission contracts from space agencies and private industry. ispace will enable clients who may want to discover, map and use the natural lunar resources.
ispace was the managing company of Team HAKUTO, one of the 5 finalists in the Google Lunar XPRIZE Competition, which was organized by the XPRIZE Foundation and sponsored by Google, called for privately funded teams to be the first to land a robotic spacecraft on the Moon, travel 500 meters, and transmit back to Earth high-definition video and images. The team developed a lunar rover named Sorato.
ispace is currently headquartered in Tokyo, Japan with offices in the United States and Luxembourg. The company’s founder and CEO is Takeshi Hakamada.
In 2023, ispace will deploy its first rover for lunar surface exploration carrying customer payloads. It will be equipped with high-definition, color photo and video, and terrain mapping capabilities, among other data acquisition technologies.
ispace aims to be a vehicle for companies on Earth to access new business opportunities on the Moon and ultimately incorporate the Moon into Earth’s economic and living sphere. Originating from the Google Lunar XPRIZE competition, in which ispace managed Team HAKUTO, one of the 5 finalists in the competition, ispace is now currently working on achieving the world’s first commercial lunar exploration program, named “HAKUTO-R” which consists of ispace’s first two lunar missions.
TeamIndus
TeamIndus (incorporated as Axiom Research Labs) is a private for-profit aerospace company headquartered in Bangalore, India. It consists of a team of professionals from various backgrounds in science, technology, finance, and media, that came together in 2010 with the aim of winning the Google Lunar X Prize competition announced in 2007.
TeamIndus’ lander was originally code-named HHK1, now called Z-01, and their rover is called ECA, an abbreviation for Ek Choti Si Asha (A Small Hope).
The founder and chief technology officer of Team Indus (India’s first space startup) had aimed to put an Indian-built rover on the surface of the Moon in competing for the international Google Lunar XPrize. But in the end, the deadlines proved to be just too difficult and Team Indus had to tell Google Lunar XPrize that they wouldn’t make the deadline. This had a lot to do with raising funds and currently, they are not very actively working on their space projects.
Firefly Aerospace
Firefly Aerospace Inc. is another private aerospace firm which is headquartered in Austin, Texas, US. Firefly is committed to providing economical and convenient access to space for small payloads through the design, manufacture, and operation of reliable launch vehicles. The Firefly team claims that they address the market’s need for flexible access to space with the simplest/soonest approach to technology selection. Firefly launch vehicles with the objectives to reduce risk, maximize reliability and minimize development time.
NASA has awarded Firefly Aerospace approximately $93.3 million to deliver a suite of 10 science investigations and technology demonstrations to the Moon in 2023. The delivery, planned for Mare Crisium, a low-lying basin on the Moon’s near side, will investigate a variety of lunar surface conditions and resources. Such investigations will help prepare for human missions to the lunar surface.
Though Firefly Aerospace missed its own 2020 deadline to launch its Alpha rocket into space, the company says it’s confident a 2021 debut is in the cards. Firefly’s desired timeline was partly derailed by the coronavirus pandemic, which has been difficult for many in the space industry.
Firefly is best known for its launch business, with the Alpha and the planned Beta. But it is also working on a lunar lander called Blue Ghost and a space utility vehicle – also known as a “space tug” — to transport satellites into unique orbits after a launch.
Firefly Aerospace said recently that it has raised $75 million in private capital. Firefly also outlined its intention to raise an additional $300 million later this year after it launches its inaugural Alpha rocket. While the $75 million finances its near-term development plans, the company wants to expand its services across the space industry.
Orbit Beyond
It is name is usually stylized as OrbitBeyond, the company builds extensible and scalable technologies for lunar exploration. Its products include configurable delivery lunar landers with a payload capacity of up to 500 kg (1,100 lb), and rovers. The company will contract for private rocket launch services.
OrbitBeyond aims to provide reliable, repeatable, and affordable payload delivery services to the Moon and cis-lunar space. They intend to make the in-space economy commercially accessible and sustainable in the near future.
They claim their product evolution is based on an extensible engineering platform; enabling commercial and scientific delivery capacity at an unmatched scale and cost.
In their mission statement, they say, “The commercial cis-lunar market is projected to grow exponentially over the next 10 years. We believe a cost reduction in accessing the cis-lunar space will spur investment into in-space infrastructure, leading to the creation of new markets for discovering & utilizing resources in space.”
On November 29, 2018, OrbitBeyond was selected to bid robotic lander contracts from NASA’s Commercial Lunar Payload Services (CLPS). OrbitBeyond has engaged former Google Lunar XPRIZE competitor TeamIndus (Axiom Research Labs) for lander engineering, Honeybee Robotics for payload integration, Advanced Space for mission management, and Ceres Robotics for surface operations. Their aim is to create collaborative and scalable spacecraft exploration platforms to support commercial market growth in the cislunar space.
On May 31, 2019, NASA announced that it had selected OrbitBeyond as one of three commercial partners to deliver NASA payloads to the Moon with its Z-01 lander in 2020 and 2021. OrbitBeyond was awarded $97 million to land NASA payloads in Mare Imbrium by September 2020, however, the company dropped out of this contract on 29 July 2019, citing its inability to launch complete the missions in the schedule. Orbit Beyond remains a CLPS contractor eligible to bid on future contracts and has currently stopped working on their space projects.
Moon Express
Moon Express state their mission statement as follows:
“Our mission is to redefine possible by returning to the Moon and unlocking its mysteries and resources for the benefit of humanity. We welcome expedition and payload partners on our commercial missions of opportunity. Our MX explorer systems are also available for charter and customer-owned missions, delivering breakthrough opportunities for scientific and commercial exploration at an unprecedented low cost.”
In August 2010, Robert D. Richards, Naveen Jain, and Barney Pell co-founded Moon Express, a Mountain View, California-based company that plans to offer commercial lunar robotic transportation and data services with a long-term goal of mining the Moon for resources, including elements that are rare on Earth, including niobium, yttrium, and dysprosium.
Beginning in 2010, Moon Express based itself at the NASA Ames Research Center. Moon Express and NASA signed a contract in October 2010 for data purchases that could be worth up to US$10,000,000.
On June 30, 2011, the company held its first successful test flight of a prototype lunar lander system called the Lander Test Vehicle (LTV) that was developed in partnership with NASA. On September 11, 2011, Moon Express set up a robotics lab for a lunar probe named the “Moon Express Robotics Lab for Innovation” (MERLIN) and hired several engineering students who had successfully competed at the FIRST Robotics Competition.
On July 12, 2018, both historic launch towers at Space Launch Complex 17 were demolished via a controlled demolition to make way for Moon Express facilities to test its lunar lander. That month, Moon Express was unable to make payroll and laid off nine employees; the employees did not receive back-pay until October 2018.
In October 2018, the company signed several collaboration agreements with the Canadian Space Agency (CSA) and a number of Canadian aerospace companies.
On November 29, 2018, Moon Express joined the Commercial Lunar Payload Services program of NASA, becoming eligible to bid on delivering science and technology payloads to the Moon for NASA.
Moon Express is also not very active these days due to lack of funding, causing it to lay off about half of its staff.
Commercial Lunar Payload Services (CLPS)
Commercial Lunar Payload Services (CLPS) is a NASA program started in April 2018, to contract transportation services to send small robotic landers and rovers to the Moon’s surface; some, but not all, to the South Polar Region known for its water ice. The goal is to search for resources that can be exploited using In-Situ Resource Utilization (ISRU) technology, and conduct lunar science in support of the Artemis lunar program. CLPS is intended to buy end-to-end payload services between Earth and the lunar surface using fixed-priced contracts.
The payloads are initially expected to be smaller instruments and investigations (less than 500 kg), but NASA will also use the deliveries for risk-reduction demonstrations to help evolve lander capabilities and capacities, and inform larger, human-class lander development. These robotic lander services, beginning as early as 2021, may be leveraged to validate precision landing technologies, cryogenic propulsion systems, and in-situ resource utilization technologies that are critical for a sustained human presence on the Moon.
NASA anticipates the need for both small and mid-size lunar landers to enable a variety of science investigations and larger technology demonstration payloads that will meet science objectives and human exploration goals. Future payloads could include rovers, power sources, science experiments and technology to be infused into the Artemis program. These services will be procured through indefinite delivery, indefinite quantity (IDIQ) contracts with a combined value of $2.6 billion.
Altair Lunar Lander
NASA has selected Altair as the name of the lunar lander the Constellation Program will use to put humans on the Moon.
The physics of spaceflight has remained unchanged, technology has matured and Altair’s missions are well beyond what the Apollo system was capable of performing. Altair will feature current technology advances in advanced computers, guidance and navigation systems, composite structures, precision landing ability, and high-efficiency propulsion systems. Additionally, each Altair lander will double the crew size of Apollo’s lunar module, and more than double the sortie mission time spent on the lunar surface. Altair will also provide an airlock to allow split crew operations and to control lunar dust.
Altair is a multi-role vehicle capable of landing crews of four astronauts anywhere on the lunar surface and supporting them for missions of up to seven days before returning them to orbit. In addition, Altair can deliver crew members to a lunar outpost facility and remain with them for up to six months, including delivery of up to 17 mt (metric tonne) of cargo to support the buildup of the lunar outpost itself. Each vehicle uses a common descent stage, with combinations of an ascent stage, an airlock, and cargo added for specific missions.
In the sortie mode, Altair can place the crew of four astronauts and up to 500 kg (1,100 pounds) of science equipment anywhere on the lunar surface and provide living quarters for the crew for up to seven days. Altair features an airlock to allow the crew to transition from its pressurized habitat to the dusty vacuum of the lunar surface. In outpost mode, Altair can deliver the four crew members to the site of a permanent lunar outpost, where it can loiter on the surface for up to 210 days, waiting to return the crew to lunar orbit at the end of their outpost stay. At the conclusion of the surface mission, the crew boards the Ascent Module.
The Altair lander also can be used to transport large cargo elements to the lunar surface. In cargo mode, the descent module is configured to autonomously land at a preselected site with up to 14,500 kg (31,900 pounds) of science equipment, lunar rovers, habitat modules, power systems, resource utilization equipment, and outpost logistics. The ability to land large cargo elements is critical to the deployment of the lunar outpost.
By the Numbers | Apollo Lunar Module | Altair Lunar Lander |
---|---|---|
Crew Size (max) | 2 | 4 |
Surface Duration (max) | 3 days | 7 days (Sortie missions), Up to 210 days (Outpost missions) |
Landing site capability | Near side, equatorial | Global |
Stages | 2 | 2 |
Overall height | 7.04 m (23.1 ft.) | 9.9 m (32.5 ft.) |
Width at tanks | 4.22 m (13.8 ft.) | 8.8 m (28.9 ft.) |
Width at footpads | 9.45 m (31 ft.) | 14.9 m (48.9 ft.) |
Crew module pressurized volume | 6.65 m3 (235 cu. ft.) | 17.5 m3 (618 cu. ft.) – crew module + airlock |
Ascent stage mass | 4,805 kg (10,571 lbs.) | 6141 kg (13,510 lbs.) |
Ascent Stage engines | 1 – UDMH-NTO | 1 – MMH-NTO |
Ascent engine thrust | 15.6 Kn (3,500 lbf.) | 24.5 Kn (5,500 lbf.) |
Descent stage mass | 11,666 kg (25,665 lbs.) | 37,045 kg (81,500 lbs.) |
Descent Stage engines | 1 – UDMH-NTO | 1 – pump-fed, throttling, LOX/LH2 |
Descent engine thrust | 44.1 Kn (9,900 lbf.) | 83.0 Kn (18,650 lbf.) |
Astrobotic’s Peregrine Lander
Astrobotic’s spacecraft, a lunar lander named Peregrine, precisely and safely delivers payloads to lunar orbit and the lunar surface. Payloads can be mounted above or below the decks, inside or outside of enclosures, and can remain attached or deployed according to their needs.
Peregrine’s avionics achieve terrestrial computing speed with high reliability. Rugged, radiation-tolerant computing enables autonomous landing and safety in the demanding space environment.
Peregrine’s structure is stout, stiff, and simple, allowing for easy payload integration. The configurable decks and enclosures accommodate payload-unique mounting and placement. Rover missions release from the underside of the deck, while BUS elements are housed inside the enclosures. Four legs absorb shock and stabilize Peregrine on touchdown.
It uses a propulsion system featuring next-generation space engine technology. Its five main engines perform all of the spacecraft’s major maneuvers, including trans-lunar injection, trajectory correction, lunar orbit insertion, and powered descent. Four clusters of attitude control thrusters maintain lander orientation throughout the mission.
Peregrine uses a high-powered, flight heritage transponder and a combination of low and medium-gain antennas to relay data between the payload customer and their payload throughout the mission. The lander-payload connection is provided via Serial RS-422 or SpaceWire for wired communications and a WLAN modem for wireless communications with deployed payloads such as rovers.
Peregrine provides 28 volts operational and heater power to payloads throughout the mission. It uses a panel of triple-junction solar cells to generate power and a space-grade lithium-ion battery to store energy. The solar panel is pointed towards the Sun whenever possible to provide continuous power generation, while the battery is utilized when the Sun is not visible or for quick discharge activities.
Peregrine’s GNC system uses heritage algorithms enhanced by recent developments in machine vision navigation. Off-the-shelf sensors and standard techniques provide reliability during cruise and lunar orbit, while Doppler LiDAR and Astrobotic’s proprietary terrain relative navigation (TRN) provide unprecedented precision during descent and landing. A scanning LiDAR can also be added to detect and avoid slopes, rocks, craters, and other hazards during landing.
The Peregrine Lander will precisely and safely deliver payloads, both into orbit around the Moon and to the surface on each mission. Payloads can be mounted above or below the decks and can remain attached or be deployed according to requirements. Peregrine avionics achieve terrestrial computing speed with high reliability. Rugged, radiation-tolerant computing enables autonomous landing. Peregrine’s structure is stout, stiff, and simple to allow easy payload integration. Its configurable decks accommodate payload-unique mountings and placement. Rover payloads can be released from the underside of the deck. Peregrine mates to the launch vehicle using a standard clamp-band. Four legs absorb shock and stabilize Peregrine on touching down. The interface options can accommodate a wide range of payload types on a single mission from companies, government, universities, non-profits, and individuals. For Mission One the payload is 90 kg (198 lb) mass capacity.
Height | 1.9m |
Width | 2.5m |
Communication | Wired and Wireless LAN |
Pay load mass capacity | 90 kg |
Navigation and Control | Radio/Doppler Sun sensors Doppler LiDAR Hazard Detection |
Fuel | MMH |
Total thrust | 3300 N |
Oxidizer | MON-25 |
Griffin Lander by Astrobotic
Griffin is a medium-class lander with flexible mounting options to accommodate a variety of rovers and other large payloads. Its autonomous sensor systems provide a safe and precise landing in even rugged and hazardous terrain, enabling it to support robotic missions such as resource prospecting, polar volatile characterization, and skylight exploration.
Griffin’s avionics achieve terrestrial computing speed with high reliability. Rugged, radiation-tolerant computing enables autonomous landing with unprecedented precision and safety in the demanding space environment.
Griffin’s aluminum frame is stout, stiff, and simple for ease of payload integration. The main isogrid deck accommodates flexible payload mounting on a regular bolt pattern. A dedicated payload adapter and optional egress ramps can accommodate rovers and other large payloads. Four legs absorb shock and stabilize Griffin during touchdown.
Griffin uses a propulsion system featuring next-generation space engine technology. Its seven main engines perform all of the spacecraft’s major maneuvers, including trans-lunar injection, trajectory correction, lunar orbit insertion, and powered descent. Four clusters of attitude control thrusters maintain lander orientation throughout the mission.
Griffin uses a high-powered, flight heritage transponder and a combination of low, medium, and high gain antennas to relay data between the payload customer and their payload throughout the mission. The lander-payload connection is provided via Serial RS-422 or SpaceWire for wired communications and an optional WLAN modem for wireless communications with deployed payloads such as rovers.
Griffin features a dedicated payload power bus to meet a wide range of payload requirements throughout the mission. The spacecraft uses a panel of triple-junction solar cells to generate power and a space-grade lithium-ion battery to store energy. The solar panel is pointed towards the Sun whenever possible to provide continuous power generation, while the battery is utilized when the Sun is not visible or for quick discharge activities.
Height | 2.0m |
Width | 4.5m |
Communication | Wired and Wireless LAN |
Pay load mass capacity | 475 kg |
Navigation and Control | Radio/Doppler Sun sensors Doppler LiDAR Hazard Detection |
Intuitive Machines Nova-C Lander
The Nova-C lunar lander draws direct heritage and lessons learned from NASA’s Project M lunar lander and experience with Project Morpheus. Project M and its terrestrial counterpart Project Morpheus were designed, developed, and tested by the NASA Johnson Space Center, Houston, TX, to demonstrate new technologies for planetary landings including autonomous hazard avoidance, precision landing, and high-performance cryogenic liquid oxygen (LOX) and liquid methane (LCH4) integrated propulsion.
In fact, the core team that was instrumental in the success of the Morpheus lander left government service specifically in order to set up the company. Intuitive Machines is leading an effort to develop a commercial Lunar Payload and Data Service to provide transit to lunar orbit, intact payload delivery to the lunar surface, and data communications and power services to assets that are in lunar orbit or on the surface.
The design is based on a dry mass of 1,500 kg (3,300 lb), a payload capacity of 100 kg (220 lb), and 200 W of power on the surface of the Moon. Nova-C is 3 m (9.8 ft) long and has a diameter of 1 m (3 ft 3 in). The plan is to catch a ride to the Moon in 2021 on a SpaceX Falcon 9 launch vehicle. After a soft landing, the Nova-C lander is capable of relocating by performing a vertical takeoff, cruise, and vertical landing. It is capable of 24/7 data coverage for its client payload. With a payload mounting surface of over 9 m2 (97 ft2), the design provides a technology platform scalable to middle-sized and large lander classes suitable for bulkier payloads.
Payload capacity to the surface of the Moon | 100 kg |
24/7 Data coverage | 250Kbps-6Mbps |
Power on the surface | At least 200 watts |
Navigation | Hazard avoidance system |
Soft touchdown | 1m/second |
Landing site capability | Anywhere on the Moon |
Payload mounting surface | Over 9m² |
OrbitBeyond’s Z-01 Lunar Lander
On May 31, 2019, NASA announced that it had selected OrbitBeyond as one of three commercial partners to deliver NASA payloads to the Moon with its Z-01 lander in 2020 and 2021. OrbitBeyond was awarded $97 million to land NASA payloads in Mare Imbrium by September 2020; however, the company dropped out on July 29, 2019, citing its inability to launch the missions on schedule. The following is a description of their proposal. Z-01 was based on the TeamIndus (incorporated as Axiom Research Labs) lunar lander formerly known as HHK1. On its maiden mission, it was to have carried up to 40 kg (88 lb) of commercial payloads.
It featured the main engine that produced 440 N (99 lbf) and sixteen 22 N (5 lbf) thrusters for finer orbital maneuvers and attitude control. Its first mission was planned to launch in Q3/2020 on a Falcon 9 rocket. They had also planned a larger Z-02 lander. The mission was targeting 29.52° N 25.68° W, just north of the crater Annegrit in Mare Imbrium.
The landing ellipse (landing footprint) for this mission was approximately 2 km × 1.9 km (1.24 × 1.18 mi). The lander would have featured automated hazard avoidance capabilities. One of the science payloads, the Lunar Ultraviolet Cosmic Imager (LUCI), is an 80 mm (3.5 in) aperture telescope to scan the sky in the near-ultraviolet to look for transient sources. The telescope was completed and tested by March 2019, so may still fly.
The Z-01 lander was also to have released a micro-rover called ECA (Ek Choti si Asha, Hindi for “A Small Hope”) that was also developed by Axiom Research Labs. ECA is a technology demonstrator tasked with visually exploring the vicinity of the landing site, to a range of at least 500 m (1,640 ft). It is a solar-powered 4-wheeled vehicle with a maximum drive speed is 6 cm/s (0.13 m/h). Its mass of less than 10 kg (22 lb) included a pair of articulated stereo cameras and a Sun sensor. Monitoring and commanding of the rover would be done exclusively via a relay through the lander. After operating until lunar sunset, the rover wasn’t expected to survive the long frigid lunar night.
Dry mass | 210 kg |
Payload capacity | 40 kg |
Propulsion | 440 N prime thruster, 16 × 22 N thrusters |
Power | Solar cells, batteries |
Fuel | Hydrazine |
Navigation | Hazard avoidance system |
Blue Ghost Lunar Lander
Blue Ghost is a lunar lander designed internally at Firefly to meet NASA’s updated requirements for a CLPS lunar lander. Despite being developed by Firefly, IAI will support the Blue Ghost lunar lander development effort as per their previous agreement on Genesis.
Firefly’s “Blue Ghost” lander was selected by NASA’s Commercial Lunar Payload Services (CLPS) program to deliver a suite of ten Payloads to the lunar surface in mid-2023, with a mission award price of $93.3 million. These Payloads will operate using lander-provided data and power resources through an entire lunar day and beyond lunar dusk in Mare Crisium. The capabilities of the Blue Ghost lander exceed those needed to complete the missions of the NASA-sponsored Payloads, and the following resources remain available for commercial use:
Payload capacity | 50 kg |
Uplink peak | 2 kbps |
Surface downlink average | 6 Mbps |
Total remaining transit payload power average | 38 W |
Transit downlink average | 2 kbps |
Peak power per payload | 196 W |
Downlink Peak | 10 Mbps |
Uplink Average | 0.2 kbps |
Total remaining surface payload power average | 300 W |
Commercial Payloads and Instruments
Sometimes NASA programs come together in support of a common goal. In this case, lunar scientists are participating in the Artemis Program. In February 2019, the agency’s Development and Advancement of Lunar Instrumentation (DALI) Program awarded 10 teams funding to mature spacecraft-based instruments for use by future lander missions, including those provided by commercial ventures by the Commercial Lunar Payload Services (CLPS) contracts. Such instruments are expected to achieve a high level of technology readiness by the time funding ends in 3 years. The DALI goals are exploration, utilization of natural resources in-situ, and lunar science.
The first instruments to be selected will have flight opportunities starting in 2021. Multiple contracts will be issued, and the early payloads will likely be small due to the limited capacity of the initial commercial landers. Also, the initial landers and rovers will be technology demonstrators of hardware for achieving precision landing and hazard avoidance, power generation, resource utilization, cryogenic fluid management, autonomous operations and sensing, mobility, mechanisms, avionics, and materials. This program requires only that a mission use American launch vehicles but not the Space Launch System (SLS). The mass of the landers and rovers can range from miniature to 1,000 kg (2,200 lb). A 500 kg (1,100 lb) lander is planned for launch in 2022.
Payload Selection Due to the short time available before the first planned flights, the first batch of science payloads are being developed in NASA facilities. Subsequent selections will include payloads from universities and industry. It is intended to make calls for payloads each year for additional opportunities. The first twelve NASA payloads and experiments were announced on February 21, 2019, and they will fly on separate missions:
- Linear Energy Transfer Spectrometer, to monitor the lunar surface radiation.
- Magnetometer, to measure the surface magnetic field.
- Low-frequency Radio Observations from the Near Side Lunar Surface, a radio experiment to measure the density of the photoelectron sheath near the surface. A set of three instruments to collect data during descent and landing on the lunar surface to help develop future crewed landers.
- Stereo Cameras for Lunar Plume-Surface Studies is a set of cameras for monitoring the interaction between the lander engine plume and the lunar surface.
- Surface and Exosphere Alterations by Landers, another landing monitor to study the effects of spacecraft on the tenuous lunar exosphere.
- Navigation Doppler LiDAR for Precise Velocity and Range Sensing is a velocity and ranging instrument to make lunar landings more precise.
- Near-Infrared Volatile Spectrometer System is an imaging spectrometer to analyze the composition of the lunar surface.
- Neutron Spectrometer System (NSS) and Advanced Neutron Measurements at the Lunar Surface is a pair of neutron detectors to quantify the hydrogen (and hence water) near the surface of the regolith.
- Ion-Trap Mass Spectrometer for Lunar Surface Volatiles is a mass spectrometer for measuring volatiles on the surface and in the exosphere.
- Solar Cell Demonstration Platform for Enabling Long-Term Lunar Surface Power, a next-generation solar array for long-term missions.
- Lunar Node 1 (LN-1) Navigation Demonstrator, a navigation beacon for providing geolocation for orbiters and landing craft.
Conclusion
This article talks about the basics of lunar lander development, some historical background, and also shares some information about the startups that are involved in the development of lunar landers. Information shared in this article shows what aspects should be kept on priority while developing a lunar lander.
It is important to note that the material used in the development, the durability of the material, reusability and sustainability, shock absorbers installed in the lander, an efficient landing system, proper guidance, and navigation system are important for a moon lander design.
All these elements must be finalized after careful consideration. In the case of Human Landing Systems (HLS), it must have the capability to accommodate the astronauts for a minimum time required by a space mission and also have enough room to carry a payload. Lessons should be learned from the previous moon missions and the landers that have been used for those missions. A lander’s structure must suit future lunar missions and space applications.
In developing a lander all other activities that will be carried out on the lunar mission should be analyzed including the in-situ resource utilization techniques that would be implemented on the Moon. In a nutshell, a lunar lander should be cost-effective, reliable, and suitable for a long-time presence on the Moon.